Four commonly-used boundary conditions in lattice Boltzmann simulation, i.e. the bounce-back, non-equilibrium bounce-back, non-equilibrium extrapolation, and the kinetic boundary condition, have been systematically investigated to assess their accuracy, stability and efficiency in simulating high Reynolds number flows. For the classical lid-driven cavity flow problem, it is found that the bounce-back scheme does not influence the simulation accuracy in the bulk region if the boundary condition is properly implemented to avoid generating non-physical slip velocity. Although the kinetic boundary condition naturally produces physical slip velocity at the wall, it gives overall satisfactory predictions of the center-line velocity profile and the vortex center locations for the Reynolds numbers considered. For the cavity flow problem, all four boundary conditions show minimal difference in the computing time needed to reach a steady state. This is surprising because the kinetic boundary condition is significantly different from the other three schemes which are designed specifically for no-slip boundary conditions. The bounce-back scheme is the most computationally efficient in updating boundary points, which is particularly attractive if there are a large number of solid bodies in the flow field. For the numerical stability, we further test the pressure-driven channel flow with or without a enclosed square cylinder. Overall, the kinetic boundary condition is the most stable of the four schemes. The non-equilibrium extrapolation scheme presents excellent stability second to the kinetic boundary condition for the lid-driven cavity flow. In comparison with other threes schemes, the stability of non-equilibrium bounce-back scheme appears to be less satisfactory for both flows.
We have investigated the accuracy, stability, and efficiency of four popular boundary conditions for LB simulation of flows with high Reynolds numbers. For the present study, the classical lid-driven cavity problem is the primary benchmark application while the channel flow around a square cylinder is used to supplement the observation of numerical stability.
It is found that all four schemes give reasonable predictions of the centerline velocity profile and the vortex position for the lid-driven cavity flow. In particular, the slip velocity given by the KBC has no significant effect on the Reynolds numbers considered (low Knudsen number). Once the non-physical slip is removed, the BB boundary condition also performs well. Moreover, the discretization accuracy is not influenced by the BB scheme in the bulk region. In fact, simulations using any of the four boundary conditions give similar orders of accuracy for the tested bulk points. The KBC shows the best stability for not only the cavity flow but also the flow around a confined square cylinder, possibly because it is able to maintain its positivity of distribution function. The NEEP scheme shows excellent stability for the cavity flow. The BB scheme shows consistent stability behavior for both flows. In the present study we find that the computational time required to reach the steady state for the lid-driven cavity flow is similar for all four boundary conditions considered. Interestingly, the KBC scheme, which is often used for rarefied gas flows, shows no significant difference in comparison to the other three boundary conditions specially designed for continuum flows. However, both the KBC and NEEP schemes need a significantly longer time to update the boundary points while the BB boundary condition, as expected, is the most computationally efficient.
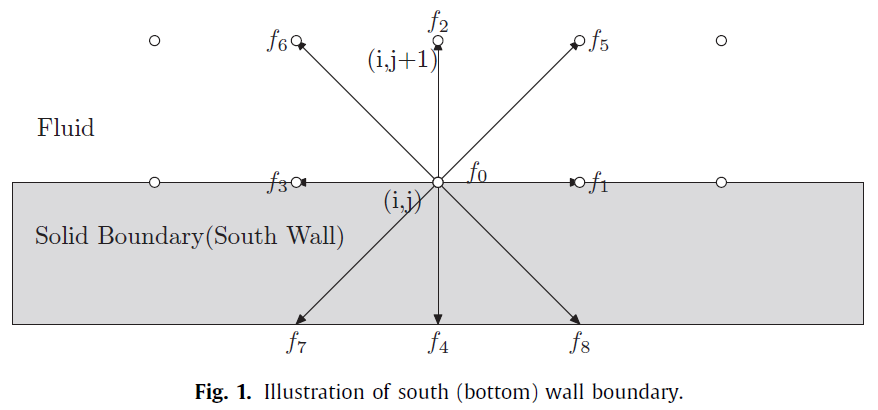